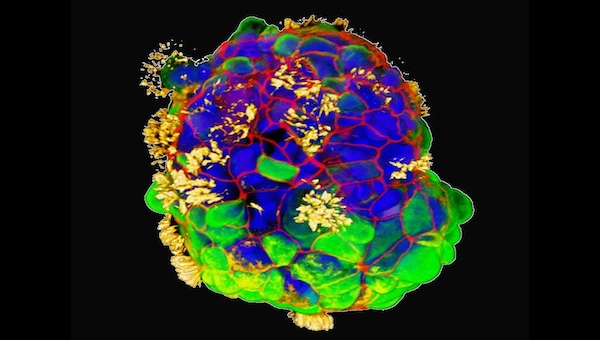
RESEARCHERS HAVE CREATED “ANTHROBOTS” OUT OF HUMAN LUNG CELLS THAT ARE CAPABLE OF MOVING INDEPENDENTLY AND EVEN HEALING DAMAGED TISSUE
ROBOTS MADE FROM HUMAN CELLS CAN MOVE ON THEIR OWN & HEAL WOUNDS
BY: PHILIP BALLORIGINAL SITE: SCIENTIFIC AMERICAN
In 2020 biologist Michael Levin and his colleagues reported that they had made “biological robots” by shaping clusters of cells into tiny artificial forms that could “walk” around on surfaces. Levin’s team argued that these entities, which it dubbed xenobots because they were made from skin and heart muscle cells of the African clawed frog Xenopus laevis , could be considered a new kind of organism. That claim became perhaps more compelling when a year later the researchers showed that xenobots could self-assemble spontaneously from the frogs’ skin cells and exhibit diverse behavior as they swam through liquid.
Some researchers argued that such behavior wasn’t so surprising in the cells of amphibians, which are renowned for their ability to regenerate body parts if damaged. But now Levin and his colleagues at Tufts University report in Advanced Science that they have made similar “robotlike” entities from human cells . They call them anthrobots.
The key to making anthrobots mobile is that, like xenobots, their surface is covered with hairlike protein appendages called cilia that wave around and propel the structures through a fluid. To actually get anywhere, the cilia all have to beat together in a coordinated fashion.
Not only can anthrobots swim, but they also seem to have distinct shapes and modes of behavior—like strains or groups within the same species of organism. And the Tufts team reports that anthrobots seem able to induce a rudimentary form of wound healing in layers of other human cells, raising the possibility of using them in medicine.
Some scientists claim that the significance of these human cell clumps, like the original xenobots, is overblown; they question whether these spontaneously forming entities can truly be considered a kind of “robot.” Some saw nothing particularly new or surprising in the idea that frog cells could form little clumps that can move. “By and large, the Xenopus embryo community who know these cells could not really see what the fuss was about,” says Jamie Davies, a developmental biologist at the University of Edinburgh in Scotland, who was not involved in Levin’s 2020 study or his recent one. He is similarly unsurprised that human cell clusters such as these will move around.
But Levin argues that the key here is a change in perspective. Rather than regarding the cell clusters as little bits of tissue that can be used to investigate human biology, they should be seen as organism-like entities in their own right, with particular shapes and behaviors that can be used as a “biorobotics platform” for medical and other applications—for example, by systematically modifying these characteristics to obtain some useful behavior such as repairing damaged tissues in the body.
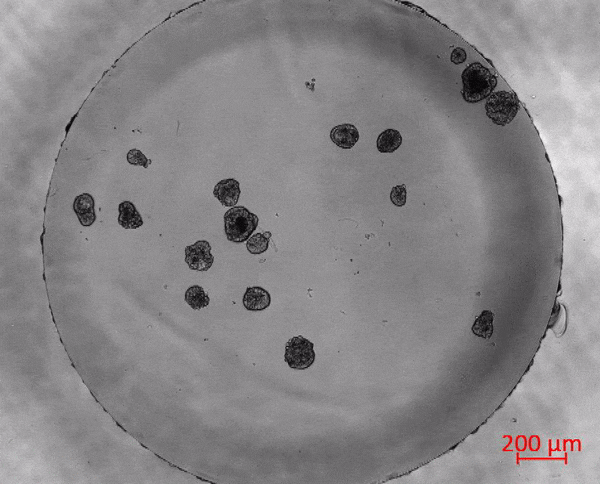
More fundamentally, Levin says, anthrobots offer a glimpse at the “morphospace” available to human cells by showing that they can spontaneously build not just the tissues and organs of the human body but also quite different structures that nature itself has never generated. “We are exploring aspects of the morphospace,” he says. “Evolution gives you a little pinpoint of variation, but there’s actually a lot more.” This ability of cells and tissues to develop different types of structures is called plasticity.
The anthrobots, each between 30 and 500 micrometers across and capable of surviving for up to two months, are made from cells taken from adult human lung tissue. This tissue naturally has cilia on its surface that wave back and forth to transport mucus, which can absorb and thus clear away debris in inhaled air. (In contrast, cilia on frog skin move mucus around to keep the skin moist.)
It is already known that this type of tissue can aggregate into ciliated clumps. Beginning in the early 2010s, several papers have reported that such aggregates, often called organoids, can be used to study lung function. In some of these the cilia point inward to a hollow interior space, as in the branches of human airways themselves. But over the past few years, researchers have also found roughly spherical airway-cell clusters (spheroids) growing with the cilia pointing out from their surface, as they do in anthrobots.
Because that previous work was concerned with making organoids as models of the human respiratory system, it didn’t include any investigation of the behavior of the cell structures. In general the studies kept the airway spheroids embedded and immobilized in a protein-rich gel called Matrigel. “Our primary goal was to develop an airway organoid system for identifying potential drug therapies to treat cystic fibrosis,” a congenital lung disease, says pathologist Walter Finkbeiner of the University of California, San Francisco, one of the authors of the earlier studies.
In contrast, Levin’s team wanted to set the spheroids free. “The tricky step is to dissolve the Matrigel gently so that you get rid of the proteins in the gel but not those that hold the bots together,” says Gizem Gumuskaya of Tufts, the new paper’s lead author. She says that two of the three previous approaches for making airway spheroids molded the clusters by making them in little wells rather than allowing them to self-assemble, as her team did, thus exploring the cells’ innate plasticity. She adds that the latter method makes the spheroids more quickly and efficiently.
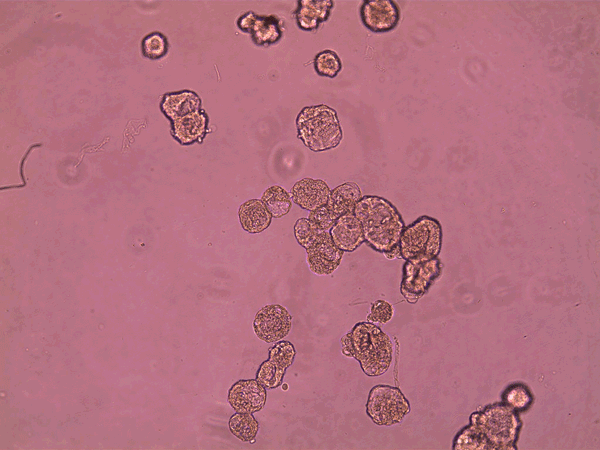
superbot, which has demonstrated the ability to encourage the growth of neurons. Credit: Gizem Gumuskaya et al., 2023 Advanced Science
The first challenge for the Tufts team will be persuading others that anthrobots are independent entities in their own right, with shapes and behaviors that the cells collectively “seek out,” rather than just somewhat random pieces of human tissue that look superficially like microorganisms.
Davies, who has previously co-authored a review article on synthetic morphology with Levin, felt there was some interest in the initial xenobot work. But he is unimpressed that the human cell clusters can “swim” with their cilia. That, he says, is pretty much inevitable if you have beating cilia once the spheroids are freed from the gel matrix. That’s just Newtonian mechanics and is purely an accidental function, he says, adding, “I cannot see how these clumps of cells with flailing cilia merit the term ‘bots.’”
The behavior of these organoids illustrates the biological functionality of the cells that comprise them, according to Salvatore Simmini and Jenna Moccia of the biotech company STEMCELL Technologies, who have also grown human airway organoids. If the coordinated motions of cilia that sweep mucus out of the airway are maintained in the organoids with cilia oriented outward, Simmini and Moccia said, the cilia will act as tiny oars propelling the cell clusters through the liquid.
Levin and his colleagues contend that these motions aren’t just random, however. After investigating the movements of hundreds of anthrobots statistically, they say that the bots seem to fall into distinct classes. In one group, the structures—small and more or less spherical—have cilia all over their surface and don’t tend to move at all. The other groups have more irregular—somewhat potato-shaped—structures that are only partly cilia-covered. They differ in having cilia that are tightly grouped in one region, leading them to swim in circular paths, or having more loosely dispersed cilia that make them move in straight lines.
The researchers say that each of these morphological and behavioral types can be considered an inherent target structure for the groups of cells—rather like the different tissue types or organs of the human body.
“What’s never been shown before is the effect these things have on other cells,” Levin adds. When the researchers set the anthrobots wandering over a flat layer of human neurons grown in a dish that had been damaged by a scratch, they found that the bots would help neurons regrow across the gap. This wasn’t just because the anthrobots supplied a passive bridge between the two edges, because small pieces of an inert polysaccharide gel didn’t have the same effect.
“We don’t know the mechanism, and that’s one of the things we’re trying to figure out,” Gumuskaya says. “But we know it’s not merely mechanical.” Levin suspects that the anthrobots are sending signals—perhaps biochemical ones—to the neurons at the scratch’s edges that encourage them to grow into the gap.
“Finding this ability was one of the first things we looked at,” Levin says. “That tells me there are probably many other things that are possible, and this is just the tip of the iceberg. This opens up the possibility of using these constructs to affect other cells [in living organisms or in a lab dish] in many other ways.” Gumuskaya hopes to look for similar “healing” behavior in models of human neurodegenerative disease, such as neuronal organoids that mimic the brain; Levin suggests that anthrobots might be used to help repair damaged retinas or spinal cords. But such ideas remain wholly speculative for now.
AWFSM CATEGORIES
Activism | AI | Belief | Big Pharma | Conspiracy | Cult | Culture | Deep State | Economy | Education | Entertainment | Environment | Faith | Global | Government | Health | Hi Tech | Leadership | Politics | Prophecy | Science | Security | Social Climate | Universe | War